Abstract
Increasing evidence suggests that an exogenous electromagnetic field might be involved in many biologic processes which are of great importance for therapeutic interventions. Pulsed electromagnetic fields (PEMFs) are known to be a noninvasive, safe and effective therapy agent without apparent side effects. Numerous studies have shown that PEMFs possess the potential to become a stand-alone or adjunctive treatment modality for treating musculoskeletal disorders. However, several issues remain unresolved. Prior to their widely clinical application, further researches from well-designed, high-quality studies are still required to standardize the treatment parameters and derive the optimal protocol for health-care decision making. In this review, we aim to provide current evidence on the mechanism of action, clinical applications, and controversies of PEMFs in musculoskeletal disorders.
Highlights
- Pulsed electromagnetic fields (PEMFs) are a noninvasive, safe and effective physical therapy, with no significant side effects.
- PEMFs provide a new alternative for the treatment of various musculoskeletal disorders.
- Further study is warranted to confirm the benefits of PEMF therapy on a variety of musculoskeletal disorders.
Graphical abstract
Pulsed Electromagnetic Fields (PEMFs) are considered to be a safe and non-invasive way to effectively treat numerous musculoskeletal disorders, such as delayed union or nonunion fractures, osteoarthritis (OA), osteoporosis (OP), osteonecrosis (ON), tendon disorders, etc. The PEMF exposure system generally consisted of a PEMF signal generator and a coil assembly with two‐coil array. The pair of coils generate magnetic field and electric field. The area between the coils is the position of the body for the person undergoing PEMF therapy. The frequency, intensity, and pulse forms are three of most crucial parameters. The frequency of electromagnetic fields used in clinical treatment is usually less than 100 Hz and the magnetic flux density is between 0.1 m T and 30 m T. All PEMF therapy devices utilize one or more of waveforms or a combination of them (such as sine square waves, triangle waves and sawtooth waves, etc.), in order to transfer the pulsed electromagnetic energy into the body. In addition, the duration of PEMF treatment is another essential factor should be taken into consideration because it is closely related to the therapeutic efficacy.
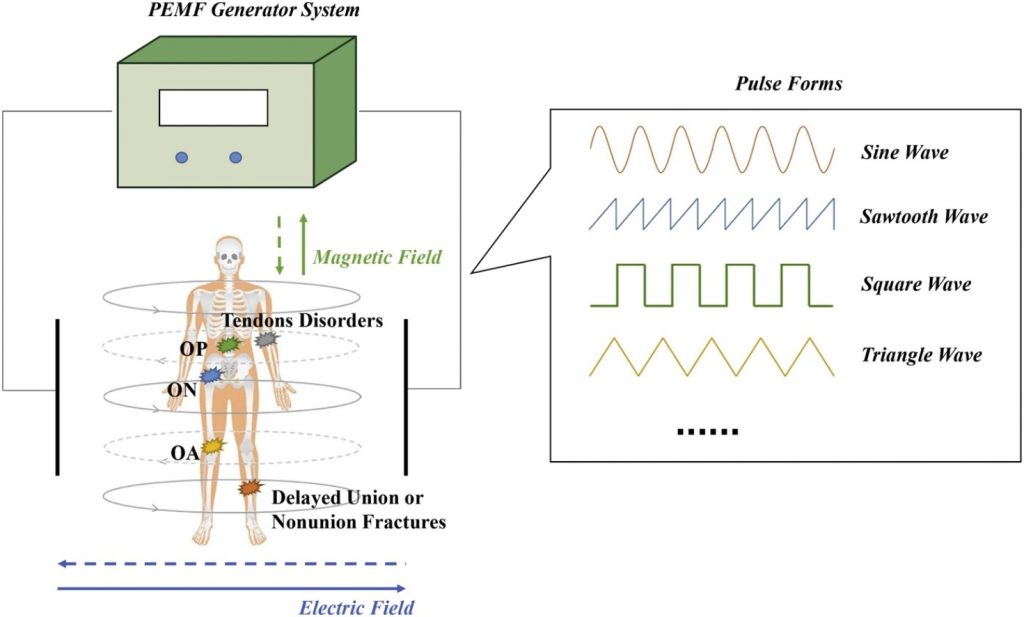
Keywords
- Pulsed electromagnetic fields
- Musculoskeletal disorders
- Application
- Therapy
- Mechanism
1. Introduction
After the design and manufacture of various types of electromagnetic signals during World War II, magnetic and electromagnetic field began to serve as therapeutic modalities [1]. In the 1950s, Yasuda et al. [2] demonstrated the existence of electric potentials in bones, including steady-state potential and stress-induced potential. Since then, researchers have generated interest in the efficacy of the electricity on bone growth and fracture repair [[3], [4], [5], [6]]. Various devices have been developed to generate electromagnetic fields for osteogenesis stimulation [7]. In 1964, Bassett et al. [8] demonstrated the effects of electric currents on new bone growth in vivo and then reported the therapeutic use of pulsed electromagnetic fields (PEMFs) in humans congenital and acquired pseudarthroses and non-unions in 1977 [9]. Then PEMF stimulation was approved by US Food and Drug Administration (FDA) to treat nonunion fractures in 1979 [10]. Subsequently, there was an abundance in the literature of reports describing the effects of PEMF therapy on bone fracture repair [[11], [12], [13], [14]].
PEMF, one of electromagnetic field therapeutic modalities, is low frequency fields with specific waveforms and amplitudes, ranging between 6 and 500 Hz [15]. It is characterized by a high rate of change (Tesla/s) that induces bioelectric currents in tissues, producing special biological effects [16]. Of note, low frequency fields have been shown to be nonionizing and nonthermal [17]. The frequency of electromagnetic fields used in clinical treatment is usually less than 100 Hz and the magnetic flux density is between 0.1 m T and 30 m T [18]. The existing PEMF has different types of waveforms including asymmetrical, biphasic, sinusoidal, quasi-rectangular, and trapezoidal [15,19]. Among them, quasi-rectangular and quasi-triangular PEMF were approved by FDA as the safe and effective treatment for fractures and their sequelae [15]. In clinical applications, Bassett’s sawtooth signal is one of the most famous pulsed magnetic field shapes, whose magnetic field changes rapidly. The rapid changes in signal strength can cause large currents in the tissue [20].
More recently, increasing evidence suggests that this noninvasive, safe and effective therapy might be as a promising adjuvant for treating various musculoskeletal disorders [[21], [22], [23], [24], [25], [26]]. However, the exact mechanism of action of PEMF at cellular and molecular level is still ongoing debate [22,27,28]. In addition, there is no standardized treatment protocols or rationale for parameter selection [29]. Therefore, extensive clinical trials and validation are still needed prior to their widely clinical application. In this review, we aim to provide current evidence on the mechanism of action (Fig. 1), clinical applications, and controversies of PEMFs in musculoskeletal disorders.
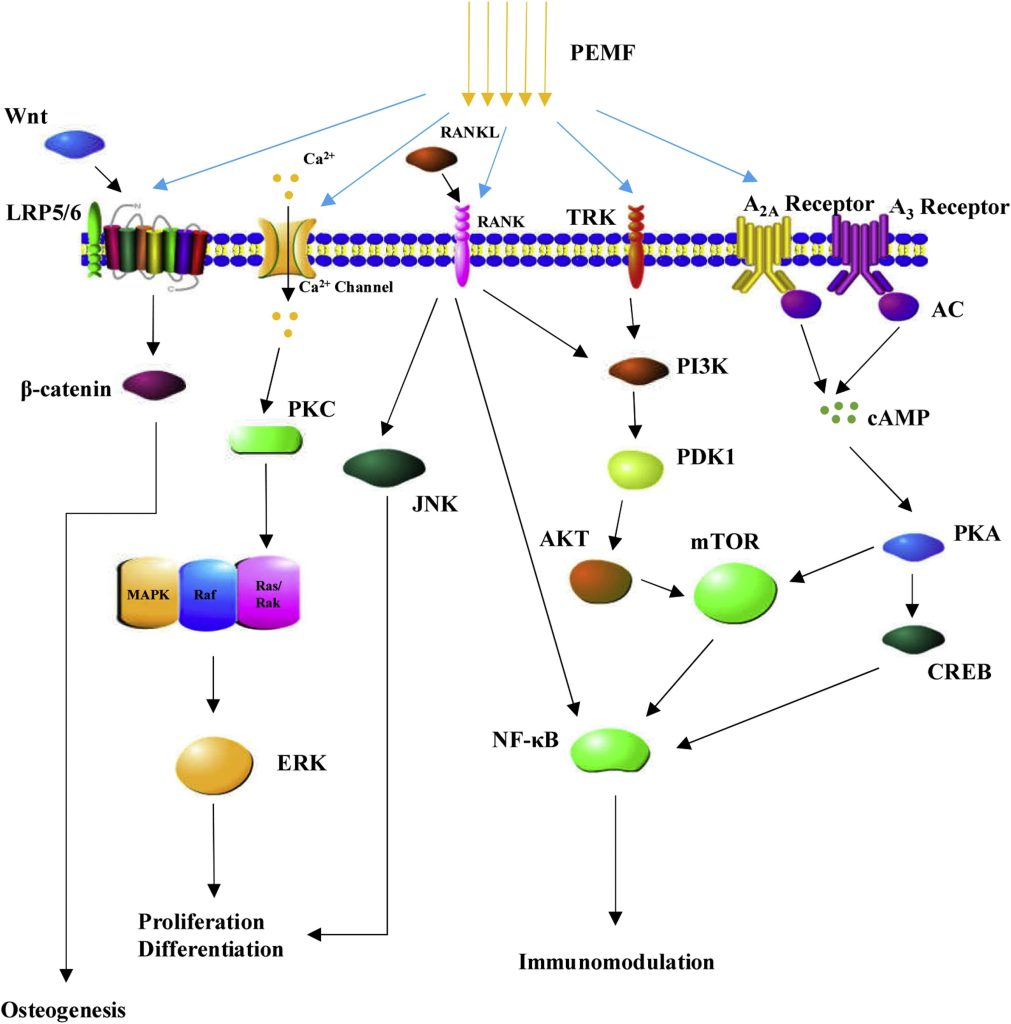
Fig. 1. The underlying molecular mechanisms of biological effects induced by PEMFs. PEMF stimulation is involved in the regulation of cell proliferation and differentiation as well as immune modulation and inflammation response through a variety of underlying molecular mechanisms.
Abbreviation: PEMFs Pulsed electromagnetic fields; PKC Protein kinase C; MAPK Mitogen-activated protein kinase; ERK Extracellular signal-regulated kinases; RANKL Receptor activator of nuclear factor kappa-B ligand; RANK Receptor activator of nuclear factor kappa-B; JNK c-Jun N-terminal kinase; TRK Tyrosine kinase receptor; PI3K Phosphatidylinositide 3-kinases; PDK1 Phosphoinositide dependent protein kinase-1; AKT Protein kinase B; mTOR mechanistic target of rapamycin; AC Adenylyl cyclase; cAMP cyclic adenosine monophosphate; PKA Protein kinase A; CREB cAMP response element-binding protein; NF-KB Nuclear factor-kappa B.
2. Mechanism of action
Over the past several decades, a collection of studies were focused on the mechanisms underlying the effects of PEMFs on biological organisms [[30], [31], [32], [33], [34], [35], [36]]. Since the currently used PEMFs are too weak to depolarize cell membranes, the biological activity of PEMFs might be associated with the amplification mechanisms that occur during transmembrane coupling [6]. The transmembrane receptors are probably sites of amplification. PEMFs were reported to affect ligand binding and distribution as well as activity of several membrane receptors including PTH [[37], [38], [39], [40]], insulin [41], IL-2 [42], IGF-2 [43], LDL [44], calcitonin [45], thereby modulating transmembrane signaling. More recently, Varani et al. also demonstrated the PEMFs exerted a strong anti-inflammatory effect on the joint environment via acting as agonist of A2A and A3 adenosine receptors [46,47]. The receptor activation can reduce the release of prostaglandin E2 (PGE2) and pro-inflammatory cytokines IL-6 and IL-8, as well as inhibit the activation of the transcription factor NF-KB [48].
A recent review summarized the underlying signaling pathways of PEMFs, including Ca2+, Wnt/β-catenin, mitogen-activated protein kinase (MAPK), fibroblast growth factor (FGF), and vascular endothelial growth factor (VEGF), transforming growth factor (TGF)-β/ bone morphogenetic proteins (BMP), insulin-like growth factor (IGF), Notch, and cAMP/protein kinase A (PKA), in bone repair [49]. Furthermore, the mammalian target of rapamycin (mTOR) pathway has also been demonstrated to be the underlying signaling pathway of PEMFs involved in bone formation [50]. Of note, PEMF exposure also affected the synthesis of growth factors such as IGF [51], BMP [52], TGF-β [53], and PGE2 [54], promoting the synthesis of extra-cellular matrix (ECM) proteins and facilitating tissue repair [6].
To date, PEMFs are still an empirically based treatment. Despite intense research, the conclusively defined mechanism of action of PEMFs remains, to a wide extent, not well elucidated. Therefore, there is still a need for further research on the underlying mechanism of action of PEMF.
3. Main clinical applications of PEMFs
3.1. Delayed union or nonunion fractures
It was reported that 5–10 % of fractures may develop delayed union or nonunion under the usual treatment [3,55]. These non-union bone fractures will not only bring along prolonged pain and disability for the patients, but also result in heavy social and economic burden [3,56]. Surgery is currently the preferred treatment for the delayed unions and nonunion fractures, but probably may bring about serious complications, such as neurovascular damage, infection, or implant-related problems [23,57,58]. As an alternative, PEMF therapy, which has attracted the attention of clinicians in recent years, has the potential to reduce the risk of these complications [22,59,60].
After PEMF therapy gain approval by the US FDA, numerous studies have reported its efficacy in treating nonunion fractures, with the overall healing rate ranging from 68 % to 90 % [13,[60], [61], [62], [63], [64], [65], [66], [67], [68], [69], [70]]. Previous in vitro and in vivo studies demonstrated that the application of PEMF therapy improved the local environment and increased expression of growth factors to facilitate the bone healing process [54,71,72]. However, indications and strategies for the treatment of nonunion fractures with PEMF vary in the literature [59,73,74]. In the opinion of most investigators, PEMF therapy would not be used until an established nonunion is diagnosed [74,75]. And other investigators used the late delayed union stage (over 6 months after fracture) as an indication for the use of PEMF [73,76]. However, a prospective randomized controlled study [59] concluded that the early application of PEMF treatment (Orthopulse® II, OSSATEC, Uden, The Netherlands, 8 h/day) can significantly improve the fracture healing rate. The average success rate in the PEMF treatment group was 77.4 % (the average duration of PEMF treatment was 4.8 months), which was much higher than that of control group (48.1 %, the average duration of sham treatment was 4.4 months). Accordingly, PEMF treatment should be considered and initiated as early as possible after diagnosis of a delayed union (at about 16 weeks after fracture).
Of note, few studies have specifically investigated effect of treatment “dose” of PEMF stimulation (The Biomet® EBI Bone Healing System (EBI, LLC (a Zimmer Biomet company), Parsippany, NJ, USA)) on the healing times of nonunion fractures. A recent study followed 1382 patients treated with PEMF stimulation and demonstrated that the average healing time was reduced by 6 days for each additional hour of daily PEMF treatment [60]. And the survival analysis showed that the median healing time of patients treated with PEMF for an average of 10 h was 35 %–60 % less than that of patients treated with PEMF for an average of 1 h per day. In addition, the study also showed that patients treated with PEMF for 9 or more hours a day healed on average 76 days earlier than those treated with PEMF for 3 or less hours a day. All these findings supported that an increase in the average daily “dose” of PEMF stimulation was associated with an acceleration in the rate of fracture healing.
3.2. Osteoarthritis (OA)
OA is one of the most common diseases of the elderly and seriously affect the patients’ life quality [77,78]. Knee is the most commonly affected joints and knee OA is a leading contributor to disability in the elderly [79,80]. Numerous therapies are available to treat knee OA, including anti-inflammatory medications, non-pharmacological treatment, and ultimately total knee replacement for advanced OA [81,82]. Current treatment is designed to alleviate pain, minimize disability and delay the progression of joint damage progression [83]. However, there are few truly effective treatments available [84].
Nowadays, various studies are focusing on the potential therapeutic effects of PEMF therapy on preventing OA development and progression, but the findings are still controversial [27,[85], [86], [87], [88]]. PEMF therapy has been demonstrated to promote stem cell proliferation and chondrogenic differentiation [[89], [90], [91], [92]]. A pre-clinical study reported that PEMFs treatment (6 h a day for 6 months), in Dunkin Hartley guinea pigs, was able to halt the progression of OA, to limit cartilage surface clefts and fibrillation, to preserve cartilage thickness and to prevent sclerosis of the subchondral bone [93]. Similarly, numerous in vitro and animal trials have shown PEMFs are beneficial for cartilage homeostasis, which contributes to preventing articular cartilage degeneration [[94], [95], [96]]. Furthermore, many randomized trials have demonstrated the potential of PEMFs to improve the symptoms of OA [97,98]. Nevertheless, a randomized, placebo-controlled study suggested that ten sessions of PEMFs (50 Hz, 30-G intensity, 90-s interval, and 30-min duration in each session) over 2 weeks had little value in reducing symptoms of knee OA [85]. In addition, one review concluded that PEMFs had no obvious effects on the management of knee OA [86]. The conflicting results might due to different study design and small sample sizes, which were not reliable enough for clinical trials [27]. Although the effect of PEMFs on knee OA remains controversial, recent studies support PEMFs as a useful complementary treatment option for knee OA [[25], [26], [27],88]. A recent meta-analysis conducted by Viganò M. and colleagues demonstrated that PEMF treatment is effective in alleviating the symptoms of knee OA in the short term, but it seems not significantly better than other conservative therapies, such as physiotherapy [99]. More reliable evidence, therefore, is still warranted to validate the efficacy of PEMF in the management of knee OA.
3.3. Osteoporosis (OP)
OP is a well-defined chronic metabolic bone disease characterized by low bone mass deteriorating microarchitecture, and increased bone fragility [28,100]. With aging of the population and longer life in worldwide, the prevalence of OP increases dramatically and results in a huge financial burden to all the countries [100]. The researchers estimated that in the future, nearly half of people over the age of 60 in developed countries will suffer from OP, 80 % of whom are postmenopausal women [101,102]. Under certain pathological conditions, the imbalance between bone resorption and bone formation leads to the abnormal bone remodeling, which contributes to various types of OP [103].
Currently, various antiosteoporosis drugs have been have proven to be effective in maintaining healthy bone mass and strength [102,104,105]. However, long term use of these drugs may lead to various potential adverse and side effects [106], such as osteonecrosis of the jaw [107], infections [108], hypocalcemia [109], increased risk of certain cancers [110], atrial fibrillation [111], and atypical femoral fractures [112]. In recent years, physical therapy for this kind of disease has got increased attention besides pharmacotherapy [113]. PEMFs, as an efficient physical therapy for various bone disorders [114,115], have been demonstrated to have the potential value in treating OP [24,28,115]. A randomized, active-controlled clinical trial [115] demonstrated that the treatment of postmenopausal OP with PEMFs at specific parameters (8 Hz, 3.82 m T, 40 min/day, and 6 times/week) and alendronate (70 mg/ week) achieved the same therapeutic effect for within 24 weeks.
A previous review has confirmed that PEMFs can significantly alleviate pain and improve quality of life in patients with primary OP [116]. Furthermore, PEMFs can not only significantly upregulate biomarkers associated with bone formation but also downregulate biomarkers associated with bone resorption [117]. However, the effects of PEMFs on bone mineral density (BMD) in patients with OP also remain controversial [[117], [118], [119]]. Garland et al. [120] showed that the BMD of patients with OP increased significantly after 3 months in the stimulated knees compared with the control group. Nevertheless, a randomized controlled single blind study suggested that no significant increase in BMD was observed after the PEMF treatment (100-Hz, 60 min per day, 3 times a week for 3 months). [119]. Similarly, Tabrah et al. [121] found no further beneficial effects of PEMFs (72 Hz, 10 h of each day of the 12 week) on BMD over an 8-year follow-up. These conflicting results might contribute to different clinical designs and parameters used by different groups [28].
3.4. Osteonecrosis (ON)
ON is considered to be a devastating disease which often involves the hip joint (femoral head) [122,123]. The most often cited risk factor for ON is high-dose corticoid administration [124,125]. In order to prevent collapse, several surgical treatments are available, such as core decompression [126], vascularized fibular grafting [127], nonvascularized bone grafting [128], osteotomy [129], and joint arthroplasty [130]. Although the surgical treatments are effective, the costs and complications of the surgery should arouse more attention [131]. If the femoral head collapses, the treatment of ON usually requires total hip arthroplasty [131,132]. Furthermore, long-term results of joint replacement in the relatively young population seem not always optimistic [133]. Therefore, prevention of ON is of great importance in patients requiring high doses of corticosteroid therapy, but there is no effective strategy to delay the progression of ON [132].
PEMFs were firstly applied by Bassett and colleagues to treat 118 patients with ON of the femoral head, which obtained the satisfactory curative effect [134]. Since then, PEMF treatment has been considered as a safe and effective treatment for patients with ON [133,135]. Massari et al. [133] performed a retrospective analysis to analyze the effects of PEMFs on patients with ON of the femoral head and concluded that PEMFs might be indicated in the early stages of this disease (Ficat stages I and II). Ishida et al. [132] used a rabbit model to evaluate and confirme the preventative value of PEMFs on steroid-induced ON. Similarly, Ding et al. [131] concluded that PEMF stimulation (15 Hz, 4 h each day for 1–8 weeks) can prevent steroid-induced ON in rats and showed the decreased serum lipid levels and increased expression of TGF-β1 might be the underlying mechanisms. In addition, Ikegami et al. [136] suggested that the underlying mechanisms of PEMF stimulation might be associated with restraint of the decreased blood flow that appears early after steroid administration.
3.5. Tendon disorders
Tendon disorders, which include both acute tendon injuries and chronic tendinopathies, are one of the most common musculoskeletal conditions and are responsible for pain and disability [137]. Generally, non-operative management is considered to be the first-line treatment, including non-steroidal anti-inflammatory drugs, ultrasound, and physiotherapy [138]. The rehabilitation times from the tendon pathologies might be extensive and the outcomes are often unsatisfactory [99]. This is partly due to the complex architecture structure of the ECM, very few of low activity tendon-resident cells, as well as the avascular nature of tendons [139]. In addition, our incomplete understanding of the molecular and cellular events underlying tendinopathy hamper the development of new and effective treatment strategies [140].
There are still a lot of disputes about the roles of inflammation in the pathogenesis of tendinopathy [138,140,141]. However, recent evidence convincingly showed that a cascade of inflammatory events, such as secretion of inflammatory mediators, infiltration of lymphocyte and macrophage, and activation of matrix metalloprotease (MMP), exert a paramount role in the etiology of tendinopathies [[142], [143], [144]]. Among various cytokines, interleukin-1β can promote tendinopathy by activating matrix metalloproteinases (MMPs), ultimately triggering catabolic degradation of ECM. Therefore, targeting the inflammatory cascade and reducing the abnormal ECM remodeling, appears to be a promising treatment for tendinopathy. Previous studies have demonstrated that PEMFs could be used to control articular tissue inflammation and stimulate anabolic pathways, that eventually lead to tissue repair [35,145]. More recently, the feasibility to use PEMFs for the management of tendon disorders has been evaluated by many studies [140,146,147]. Gehwolf et al. [140] provided evidence that in vitro treatment of tendon-derived cells with PEMFs might limit their catabolic effects of a pro-inflammatory stimulus by Il-1β, thereby resulting a more tissue reparative state. Other study at cellular level has also shown that PEMFs might be effective in the modulation of gene expression, cell proliferation and cytokine production in human tendon cells [148]. Interestingly, PEMF was found to have no effect on gene expression of tenocytes in standard culture medium, however, it has a positive role in enhancing their gene expressions under an inflammatory condition [149]. This evidence suggests that PEMF might be suitable to treat tendon disorders associated with inflammation. Girolamo et al. [147] evaluated different biological effects exerted by different PEMF intensity, duration and length of exposure, and concluded that repeated 1.5 mT-PEMF treatment showed the best biological effects.
Moreover, the failure of tendon healing may also be attributed to the incomplete restoration of tendon body and lack of osteointegration at the level of the enthesis [150]. Therefore, the enhancement of tenogenic process during tendon regeneration and the improvement of enthesis reconstruction are effective methods to improve tendon repair. Previous studies showed that PEMF exposure on human tendon increased the expression of collagen type I, as well as production of IL-10 and VEGF, which can promote the healing process of tendon [147,148]. Of note, the application of mesenchymal stem cells (MSCs) in tendon healing and regeneration is still a debate but novel therapies [150,151]. A controlled laboratory study demonstrated that PEMFs have little effects on the proliferation of human tendon stem cells (hTSCs) but enhance the expression of stem cell marker [151]. Therefore, the application of PEMFs might open up new perspectives for tendon regeneration
3.6. Other conditions
Including the main applications mentioned above, PEMFs have also been shown to be beneficial in the treatment of lateral epicondylitis [152,153], fibromyalgia [154,155], degenerative disc disease [[156], [157], [158]], musculoskeletal chronic pain [21,159], and nerve regeneration [160,161]. As can be seen, the indications for PEMFs in the treatment of musculoskeletal disorders will continue to expand with time and experience.
3.7. Limitations and adverse effects
Although PEMFs show satisfactory therapeutic effect on various musculoskeletal conditions, there still several issues remain unresolved. Firstly, numerous studies have focused on the underlying mechanism of action of PEMFs, but deeper mechanisms are still unknown [27,28,72,94,132,162]. Secondly, there are no standardized clinical protocols or rationale for parameter selection. Different parameters of PEMF, such as frequency, intensity and exposure time, were manipulated in different clinical and experimental studies [27,28]. For one specific disease, the optimal parameters of PEMF therapy are still not known. As is the case with dosage of medicines, the correct physical parameters of PEMF should be found in each specific clinical case. In addition, the small samples sizes used in many studies remains as a limitation [59,88,163]. So, it is necessary that rigorously designed high quality, large-scale randomized controlled trials with long-term follow-up are warranted to confirm the effects of PEMFs on patients with musculoskeletal disorders.
PEMFs are generally considered to be a safe and effective technique for patients and study participants if appropriate precautions are taken [164]. However, it is still necessary to assess whether PEMFs pose any risks to the health of operators and patients in routine clinical use. Numerous safety exposure guidelines and expert opinions from competent authorities and expert groups have provided that exposure to electromagnetic fields might have potential adverse effects on the brain and peripheral nervous system, cardiovascular system, cognitive and vestibular function [[165], [166], [167]]. Moreover, previous studies have reported that electromagnetic fields exposure were associated with depression and suicide and neurodegenerative diseases among utility workers [[168], [169], [170], [171]]. Of note, recent studies demonstrated that long-term exposure to man-made electromagnetic fields may led to DNA damage, which is associated with an increased risk of seizures, syncope, cancer, neurodegenerative diseases, reproductive declines, etc. [[171], [172], [173]]. For the sake of security, therefore, high attention must be paid to the potential adverse effects of long-term PEMFs in further studies.
4. Conclusion
Based on the currently positive findings of the emerging published studies, PEMFs show great potential to become a stand-alone or adjunctive treatment modality for treating musculoskeletal disorders, due to their noninvasiveness, safety and efficacy. Considerable studies have explored the underling cellular and subcellular mechanisms of PEMF stimulation on various musculoskeletal diseases, providing a molecular basis for extending its clinical application. However, several issues remain unresolved, such as the unknown deeper mechanisms and optimal parameter selection. Accordingly, prior to their widely clinical application, further researches from well-designed, high-quality studies are still required to clarify the deeper mechanisms, standardize the treatment parameters and derive the optimal protocol for health-care decision making. In summary, with adequate patient selection, appropriate indications, and homogeneous therapeutic strategies, PEMFs could exert a potent role for treating certain musculoskeletal disorders in the future.
Author contributions
HZH, WBY and QWZ wrote the manuscript. WC, YBZ and WJL revised the full paper. BCW, ZWS and YZZ reviewed and edited the paper. All authors read and approved the paper.
Ethics approval and consent to participate
None.
Consent to publish
None.
Data availability
Not applicable.
Funding information
None.
Declaration of Competing Interest
The authors report no declarations of interest.
SOURCE FROM SCIENCEDIRECT